Essential Macronutrients: Sulfur (S)
So far we’ve covered he big three macronutrients: nitrogen (N), phosphorus (P), and potassium (K) — the famous NPK. Today we’ll cover a lesser-known, but also important nutrient for plants: sulfur (S).
It may surprise you that sulfur is an essential macronutrient — most people think of sulfur as the pure yellow powder in long-ago school science labs, or maybe as a component of hydrogen sulfide (H2S), or ‘rotten egg gas’. Yet sulfur has a very important role in both animal and plant biochemistry, which we shall see.
Availability to Plants
Temperate soils contain 0.005 – 0.04% total sulfur, and those with more organic matter tend to hold more sulfur. Total sulfur in dry plant tissue is around 0.2 – 0.5%.
Sulfur exists in soil in both organic (with carbon) and inorganic (without carbon) forms.
The inorganic form is mostly as sulfate ions (SO42-), and it is in this form that sulfur is mostly absorbed by plants.
In dry soils, sulfate ions combine with calcium (Ca2+), magnesium (Mg2+) and sodium (Na+) ions to form salts [calcium sulfate (CaSO4), magnesium sulfate (MgSO4) and sodium sulfate (Na2SO4) respectively]. (Magnesium sulfate is also known as ‘Epsom salt’, after a saline spring in Epsom, Surrey, England.)
These salts can build up to high levels in the upper soil layer under dry conditions. In wet conditions however, sulfate ions are either loose in the soil water or bind to colloids. Sulfate ions in these conditions enter an equilibrium between soil water and colloids as does phosphorus in the form of phosphate, and becomes exchangeable sulfur. Sulfate’s ability to adsorb to colloids decreases with increasing soil pH and increases with increasing clay content. Large amounts of loose sulfate ions may leach in the increased presence of water.
Some sulfur enters the soil via the atmosphere as sulfur dioxide (SO2). This is a natural part of the sulfur cycle, but burning of fossil fuels has increased atmospheric levels of this gas significantly. (I’d like to cover the sulfur cycle in more detail in a future post.)
Organic forms of sulfur can come from manures and dead plant and animal material, and is made available to plants via microbial activity. In anaerobic conditions (absence of oxygen), this organic sulfur is metabolised (’eaten’ and converted) by chemotrophic (’nourishment from chemicals’) sulfur bacteria into hydrogen sulfide (H2S). If anaerobic conditions persist, this hydrogen sulfide is then further converted into elemental sulfur (S) by the photosynthetic green and purple bacteria. (These bacteria utilise H2S instead of H2O (water) in the presence of photons to make glucose, producing pure sulfur rather than oxygen gas (O2) as the by-product. These anoxygenic photosynthesisers were Earth’s first photosynthesisers and were very briefly mentioned in A Crash Course in Photosynthesis .)
In aerobic conditions (presence of oxygen), H2S auto-oxidises into sulfate (SO42-) and is also converted by the same chemotrophic sulfur bacteria mentioned above into sulfuric acid (H2SO4). Elemental (pure) sulfur (S) can also be converted into sulfuric acid by lithoautotrophic (’self-nourishment from rocks’) bacteria of the genus Thiobacillus.
The sulfuric acid molecules dissociate into H+ and SO42- ions in the soil water. The SO42- ions becomes available to plants and the soil becomes more acidic from the additional H+ ions. (This is why sulfur is traditionally applied to alkaline soil to lower the pH.)
Unlike phosphate, sulfate uptake by plants is not pH sensitive in the range in which roots are typically exposed.
Sulfur-Containing Amino Acids
Cysteine and methionine are the two most important sulfur-containing amino acids in plants. As plants must make these for themselves, it is vital that sulfur be available, hence why this is a macronutrient.
In extremely simplified terms, sulfate reacts with ATP to form cysteine, the first stable sulfur-containing organic (contains carbon) compound made by a plant. Through still more reactions, cysteine is converted to methionine.
Both cysteine and methionine participate as free acids in other reactions — cysteine is a precursor in the making of vitamins and antioxidants, while methionine is a precursor in the making of glutathione (an antioxidant) and the plant hormone ethylene.
Both, as amino acids, are also essential in the formation of proteins and enzymes (which are also proteins). But cysteine (not methionine) has an additional role in that it helps proteins fold into their correct shapes via disulfide bridging — an S-S bond that forms a bridge between two molecules.
Here are two cysteine molecules joined together via an S-S bridge:
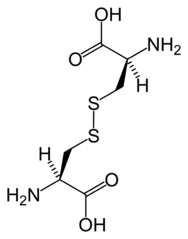
Attribution: No machine-readable author provided. Benjah-bmm27 assumed (based on copyright claims). / Public domain
This following image represents a string of amino acids — a protein in other words. Cysteine molecules along this protein will form disulfide bridges with other cysteine molecules in other locations along the molecule, causing the protein to fold in a particular way.
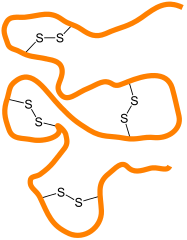
Attribution: Jü / CC0
Different proteins will fold in their own unique ways, determined by where cysteine amino acids in each protein are in relation to each other.
Correct protein folding is extremely important in biochemical reactions — as enzymes are proteins, and many reactions depend on enzymes, those reactions simply won’t occur, or will produce faulty end products, if the enzyme’s shape is ‘wrong’. Cystic fibrosis and sickle cell anaemia in humans are classic examples of incorrect protein folding. These are both genetic diseases, each caused by just one faulty protein not folding correctly [cystic fibrosis transmembrane conductance regulator (CFTR) protein in sufferers of cystic fibrosis, haemoglobin in sufferers of sickle cell anaemia].
Sulfur Deficiency Symptoms
A deficiency of sulfur will prevent cysteine and methionine synthesis, which in turn prevents crucial proteins and enzymes from forming.
Chloroplast formation is affected, which reduces photosynthetic activity and sugar production. A lack of essential proteins and enzymes in general leads to stunted growth overall. The growth of shoots is more affected than the growth of roots, and plants tend to be rigid, brittle, and with thin stems.
Unlike nitrogen, potassium and phosphorus deficiencies, a sulfur deficiency appears in the youngest leaves first. This implies that sulfur is not as mobile in plants as the other three macronutrients — sulfur here is ‘locked away’ in proteins and a plant must rely on uptaking sulfate from the soil for continual protein production.
The topmost affected leaves will change colour from a light green to become more and more yellow as chloroplasts degrade, followed by leaves further and further down. The internode length (the distance between nodes along a stem or branch) may decrease as growth decreases.
Sulfur Toxicity Symptoms
Plants are not sensitive to high levels of sulfate in the soil, except where there are high concentrations in saline soils. Here, the effects of reduced growth rate and dark green leaves are not specific to a sulfur excess so much as they are more typical of salt-affected plants in general.
Plants can absorb some sulfur dioxide (SO2) through their leaves without harm, but high concentrations such as around industrial areas can be toxic. SO2 dissolves in the moist leaf tissue and forms sulfurous acid (H2SO3), which dissociates into H+, HSO3- and SO32- ions. These ions, along with SO2, disrupt chloroplast membranes and interefere with photosynthesis processes generally. This toxicity presents as necrotic (localised, dead) tissue in leaves.
About the Author

BSc(Hons), U.Syd. - double major in biochemistry and microbiology, with honours in microbiology
PhD, U.Syd - soil microbiology
Stumbled into IT and publishing of all things.
Discovered jujube trees and realised that perhaps I should have been an agronomist...
So I combined all the above passions and interests into this website and its blog and manuals, on which I write about botany, soil chemistry, soil microbiology and biochemistry - and yes, jujubes too!
Please help me buy a plant if you found this article interesting or useful!