Essential Macronutrients: Phosphorus (P)
Of the six essential macronutrients a plant needs, the big three are nitrogen (N), potassium (K), and phosphorus (P), our subject for today.
Availability to Plants
Elemental phosphorus (P) makes up 0.02–0.15% of soil, and primarily exists as hydrogen phosphate (HPO42-) and dihydrogen phosphate (H2PO4-) ions. These are both simply called phosphates (orthophosphate ions to be more specific), and are anions (negatively-charged ions).
Australia’s very old soils are typically low in total P. Phosphorus is not mobile in soil and is best applied close to the roots, but as phosphorus tends to remain in the upper soil there is also a risk of run-off during heavy rainfall.
As with soil potassium ions, the phosphate ions exist in three soil fractions: those dissolved in the soil water, those attached to colloids and which are in equilibrium with the soil water, and those locked away in soil minerals until released slowly due to weathering.
We learnt earlier that pH is determined by the concentration of hydrogen ions (H+), and that soil pH determines the availablility of plant nutrients. With regard to phosphate availability, soil pH further determines the ratio of HPO42- to H2PO4-.
HPO42- is almost absent at pH 5 (acidic) but both ions are in roughly equal amounts at pH 7 (neutral). On the other hand, H2PO4- is the one to decrease in concentration as pH increases (and the soil becomes more alkaline).
This is because the two ions enter an equilibrium dictated by the presence of hydrogen ions (H+):
HPO42- + H+ ↔ H2PO4-
In the presence of more H+ (a more acid pH), HPO42- ions in the soil will take up those H+ ions to form H2PO4- ions. (And the equation shifts to the right.) The concentration of HPO42- drops to virtually zero in very acidic soil as they have mostly formed H2PO4- ions.
In more alkaline environments however, where there are less free-floating H+ ions available, H2PO4- ions are more likely to give up H+ ions into the soil water and form HPO42- ions. (The equation shifts to the left.) However, this doesn’t necessarily mean that those HPO42- ions become more available, especially in very alkaline soils as we’ll see shortly.
Overall, phosphorus solubility is very pH-dependent in soils. If most of the available phosphates are attached to colloids, an increase in pH will help those phosphates detach and enter the soil water. pH also becomes a factor in the rhizosphere (the region immediately surrounding the roots), which can be as much as one unit of pH higher or lower than the surrounding soil. This really needs to be the subject of a separate post, but this difference in pH is due to whether nitrate or ammonium is the dominant nitrogen nutrient, which influences whether anions (negatively-charged ions) or cations (positively-charged ions) are uptaken in higher amounts, which in turn determines the amount of H+ ions near the roots, which determines pH. In short, an increase in rhizosphere pH should enable more phosphate to detach from colloids and become more available to roots.
Going back to the highly alkaline soils though, such soils typically have high levels of carbonate (CO32-) and bicarbonate ions (HCO3-). Soluble phosphate availability decreases in calcareous soils [those containing calcium carbonate (CaCO3)], as phosphate and calcium ions (Ca2+) combine to form calcium phosphate [Ca3(PO4)2], which is not very soluble in water. Soluble phosphate availability also decreases in alkaline soils generally as phosphate preferentially binds to soil colloids when there are very few H+ ions available. In both cases, phosphate ions are inaccessible, either as calcium phosphate or on soil colloids.
Similarly, phosphates form insoluble compounds with iron and aluminium in very acidic soils which lowers their availability in those. [Iron phosphate can exist as iron (ferric) phosphate (FePO4) or iron (ferrous) phosphate (Fe3(PO4)2). Aluminium phosphate is AlPO4.]
An interesting, biological (biotic), route by which phosphate becomes available to plants is via the hyphae (long filaments) of endotrophic (literally ‘nourishment from internally’, but here meaning ‘living inside root cells’) mycorrhizae (plural form of mycorrhiza, ‘root fungus’). In exchange for sugars from photosynthesis, mycorrhizal fungi provide the plant with phosphorus (and often water and other nutrients). The long, exploring hyphae of the fungi are in essence an extension of a plant’s roots, but finer and further in their reach.
Organic Phosphate Compounds
Phosphorus is uptaken as inorganic phosphates, ie in compounds that do not contain carbon — hydrogen phosphate (HPO42-) and dihydrogen phosphate (H2PO4-). Once inside a plant however, phosphorus rapidly becomes incorporated into organic phosphates, ie ones that do contain carbon. Many of these are the phosphorylated sugars and alcohols. Sugars and alcohols contain only carbon (C ), hydrogen (H) and oxygen (O); phosphorylated ones have a phosphorus atom as well. These compounds are mostly intermediaries in many metabolic reactions — they don’t have an end purpose per se, but rather are the intermediate products of reactions that then feed into other reactions until some end product is finally made.
By way of example may I refer you yet again to this image, and specifically the left-hand side where compounds with the words ‘phosphate’ and ‘phospho’ feature heavily. None of these are standalone molecules; they are all intermediaries in a series of reactions by which glucose (a simple sugar) is ultimately converted into pyruvate (a compound essential in cellular respiration).
And just by way of illustration as to what a phosphorylated anything looks like, let’s take the first two molecules in that reaction: glucose and glucose 6-phosphate. This is glucose, comprised only of carbon, hydrogen and oxygen. Each carbon atom is numbered 1 to 6 so chemists and biochemists can refer to specific ones:
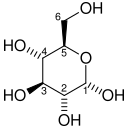
Attribution: Me, Stanislaw Gackowski (Soeb)updated by Rubber Duck (☮ • ✍) [Public domain]
And this is a phosphorylated glucose, glucose 6-phosphate (here a chemist/biochemist can tell immediately from the name that the phosphate ion is attached to carbon number 6, and derive its structure accordingly):
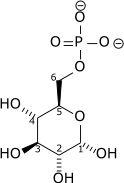
Attribution: Richard Wheeler (Zephyris) [Public domain]
Adenosine Triphosphate (ATP)
Phosphorylation is a very important process in biochemistry, and one very important end product from phosphorylation is adenosine triphosphate (ATP). ATP was first mentioned in the discussion on nitrogen, and here it is again to show, this time, the three phosphorus atoms/three phosphate ions:
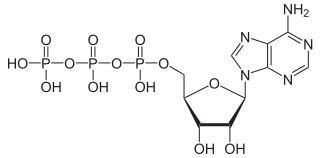
Attribution: NEUROtiker [Public domain]
ATP is the energy carrier that powers cellular processes, and is absolutely essential to all life no matter how simple or complex.
Not only is nitrogen essential for the formation of this molecule, but so too is phosophorus.
DNA and RNA
Another place where phosphorus end up is in the backbone of DNA and RNA. Here is DNA (RNA is more or less a single-stranded version of this) showing the phosophorus atoms in yellow. Each phosphorus has four oxygens attached to it, making this a phosphate group.:
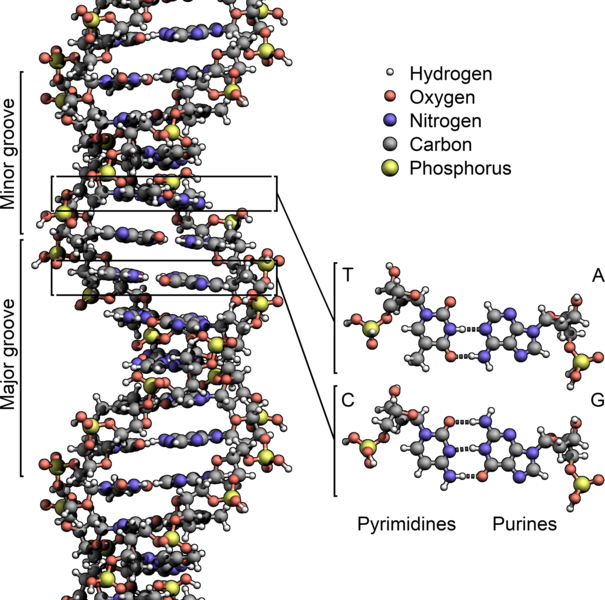
Attribution: Zephyris [CC BY-SA (https://creativecommons.org/licenses/by-sa/3.0)]
DNA and RNA are essential for the formation of proteins and enzymes, which are vital for cellular processes, growth and reproduction.
Phosphorus Deficiency Symptoms
Plant deficiencies will generally occur once the concentration of P in dry weight matter falls below 0.2%.
Having seen phosphorus’ importance in ATP, DNA and RNA, it should be no surprise that phosphorus-deficient plants show stunted growth. The dry matter shoot:root ratio is also typically low. P-deficient fruit trees often exhibit a reduced growth rate of new shoots and a poor development and opening of buds. Fruit and seed yields are often low and of poor quality. New leaf size and numbers of new leaves may be small.
As with nitrogen and potassium, phosphate too is highly mobile in plants. Again as with nitrogen and potassium, symptoms first appear in older leaves, as phosphate moves not only up into young leaves, but down as well into growing root tips. Older leaves stripped of phosphates take on a dark green colour, even becoming blue-green in some species. Fruit tree leaves may be tinged with a brownish colour. This colouration is due to a buildup of excess carbohydrates that are converted into purplish pigments called anthocyanins.
Phosphorus Toxicity Symptoms
Plants will show stunted growth, and even die, in the presence of too much phosphorus too. Toxicity is more pronounced within a pH range of 5 to 7, where phosphates are more likely to be in a soluble state in the soil water. Large applications of poultry manure, higher in phosphorus than other manures, can cause toxicity especially on sandy soils which cannot absorb phosphate ions as readily as more colloidal soils.
Excess phosphate in soil will form insoluble compounds with iron (iron phosphate) and zinc [zinc phosphate, Zn3(PO4)2], leading to deficiencies in these.
Phosphates are not very mobile in soil. Plants will not uptake any more phosphate than they need, and thus additional applications will tend to accumulate in the upper soil, exacerbating the onset of toxicities. As phosphates tend to attach to soil particles, heavy runoff and associated erosion can send excess phosphorus to river or other water systems. Phosphorus, along with nitrogen, is a limiting nutrient in water, thus phosphate- and nitrogen-rich fertilisers that enter waterways will produce algal blooms.
Phosphorus is very much a nutrient for which ‘if some is good then more is better’ definitely does not apply.
About the Author

BSc(Hons), U.Syd. - double major in biochemistry and microbiology, with honours in microbiology
PhD, U.Syd - soil microbiology
Stumbled into IT and publishing of all things.
Discovered jujube trees and realised that perhaps I should have been an agronomist...
So I combined all the above passions and interests into this website and its blog and manuals, on which I write about botany, soil chemistry, soil microbiology and biochemistry - and yes, jujubes too!
Please help me buy a plant if you found this article interesting or useful!