Endosymbiotic Origin
Backstory: Of Prokaryotes and Eukaryotes
Prokaryotes
Prokaryotes (pronounced ‘pro-carry-oats’) comprise the two Domains Bacteria and Archaea. Prokaryotes are defined as unicellular organisms without membrane-bound nuclei, and contain a single circular chromosome.
Cyanobacteria
Many prokaryotes can photosynthesise via compounds known as bacteriochlorophylls. They are all, with one exception, anoxygenic photosynthesisers, in that they do not produce oxygen as a by-product.
Cyanobacteria are that one exception, being oxygenic photosynthesising prokaryotes and producing oxygen as a by-product. They are also the only chlorophyll-containing prokaryotes. This is significant, as we’ll see below.
Eukaryotes
Eukaryotes (pronounced ‘you-carry-oats’) belong to a single Domain, Eukaryota, and are defined as organisms with membrane-bound nuclei. These are ‘everything else’ and include unicellular and multicellular organisms. Yeasts, fungi and protozoa are as much eukaryotes as multicellular plants and animals. (This is why fungal infections are very hard to treat, as their cellular structure is similar to ours, and what kills them has the potential to also kill or severely harm us.)
Eukaryotes have tightly wound chromosomes within their nucleus. There’s no correlation between an organism’s size or complexity and its number of chromosomes. For example, the Australian jack jumper ant (Myrmecia pilosula) has the smallest number of all eukaryotes, with males having one chromosome and females having two. Yet the much smaller (and simpler) protozoan Oxytricha trifallax has the largest number of all organisms, with 16,000 (very short) chromosomes! Humans have 46 and the jujube tree has 24. A species of Adders-tongue fern, Ophioglossum reticulatum, has 1,260!
Chloroplasts
All eukaryotic cells contain organelles — ‘little organs’, with the nucleus and mitochondrion the two best-known examples. Nuclei and mitochondria are common to all eukaryotes, but only the photosynthesising eukaryotes have the photosynthesising organelles called chloroplasts.
A chloroplast looks like Fig. 1 below (and we’ll be picking this apart in more detail later):
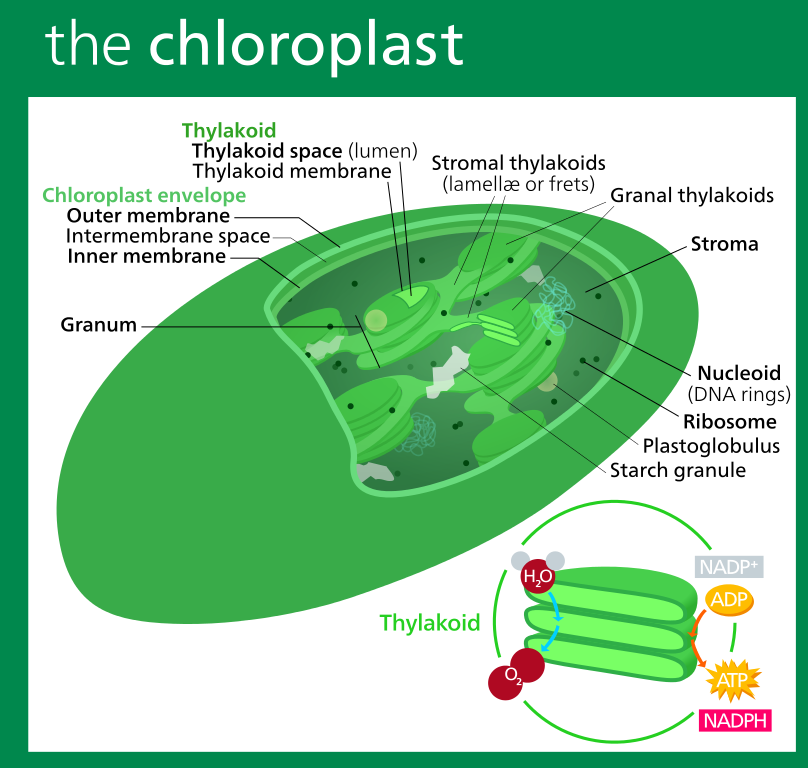
Attribution: Kelvinsong, CC BY 3.0 , via Wikimedia Commons
There are typically many chloroplasts per cell (Fig. 2):
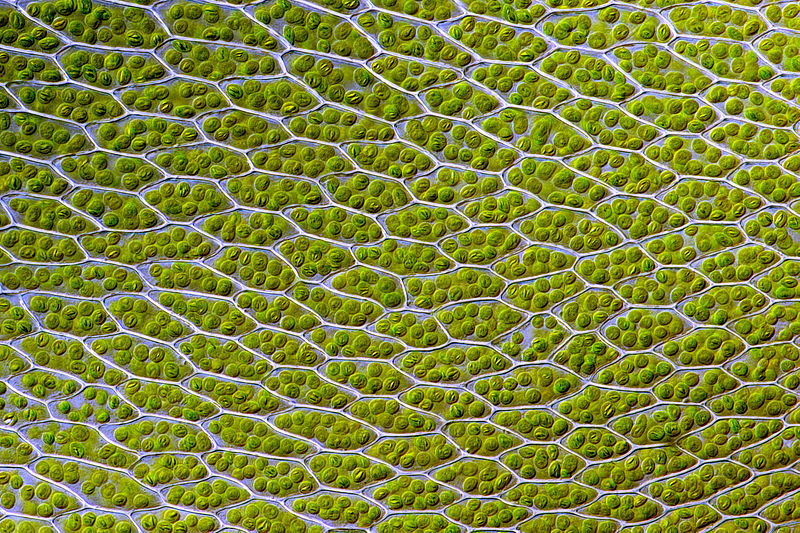
Attribution: Des_Callaghan, CC BY-SA 4.0 , via Wikimedia Commons
Chloroplasts are green as they contain chlorophyll, the actual green pigment which absorbs photons and initiates photosynthesis. Thus any green part of a plant, even if a young branch, both contains chloroplasts and is able to photosynthesise.
Symbiogenesis, or the Endosymbiotic Theory
Meaning
‘Symbiogenesis’ comes from the Ancient Greek σύν, syn, ‘together’, βίος, bios, ‘life’, and γένεσις, genesis, ‘origin/birth’: ‘life coming together to create new life’).
The theory of symbiogenesis, also known as the endosymbiotic theory, explains the origin of eukaryotic cells from prokaryotic ones. The prefix ‘endo’ in ‘endosymbiotic’, which also comes from Ancient Greek (ἔνδον, éndon, ‘inner; internal’), signifies that this symbiosis involved one organism being completely inside the other.
Development of the Theory
Hugo von Mohl was the first to describe chloroplasts in 1837, though he called these Chlorophyllkörnen (‘grain of chlorophyll’). Andreas Franz Wilhelm Schimper named these Chloroplastiden (‘chloroplastids’) in 1883, and Eduard Adolf Strasburger named these Chloroplasten (‘chloroplasts’) in 1884.
Also in 1883, Schimper observed that chloroplasts — found only in eukaryotic photosynthesising cells — closely resembled cyanobacteria, and that chloroplasts were also self-replicating. He put forward (in a footnote) the idea that green plants had arisen from a symbiosis of two organisms.
The Russian botanist Konstantin Mereschkowski was influenced by Schimper’s work, and in 1905 proposed further that cell nuclei and chloroplasts descended from a bacterium that had formed a symbiosis with an amoeba.
The French scientist Paul Jules Portier suggested in 1918 that mitochondria also came from a distant symbiosis, and Ivan Emanuel Wallin earned the nickname ‘Mitochondria Man’ from all his work on the subject in the 1920s.
Finally, the electron microscopy work of Hans Ris and especially Lynn Margulis in the 1960s established symbiogenesis as the most accepted theory to this day, as to the origins of both chloroplasts and mitochondria.
One Model for the Theory of Symbiogenesis
Fig. 3 shows one model for the endosymbiotic theory. Here, a prokaryote ancestor first developed a cell membrane around its DNA, thus creating a nucleus and becoming the first eukaryote. This now-ancestor to all eukaryotes then engulfed an aerobic (oxygen-using) prokaryote, which for whatever reason wasn’t digested, but remained inside the eukaryote as an independently-living entity.
It is highly significant that this prokaryote was aerobic, as oxygen is a very strong electron-acceptor. Using oxygen enabled this organism to produce huge amounts of energy compared to what anaerobic prokaryotes could generate from other electron-acceptors such as iron, nitrate, sulfate, and carbon dioxide.
This high energy output enabled the eukaryote host to thrive, and eventually the prokaryotic symbiont became a mitochondrion, still the energy factory of all eukaryotic cells today.
Some time later a eukaryote-with-prokaryote ancestor similarly engulfed a cyanobacterium, and that cell became the ancestor of all plants and algae, with the cyanobacterium symbiont becoming the ancestor of all chloroplasts. (Animal cells have mitochondria only, while plant and algae cells have both mitochondria and chloroplasts.)
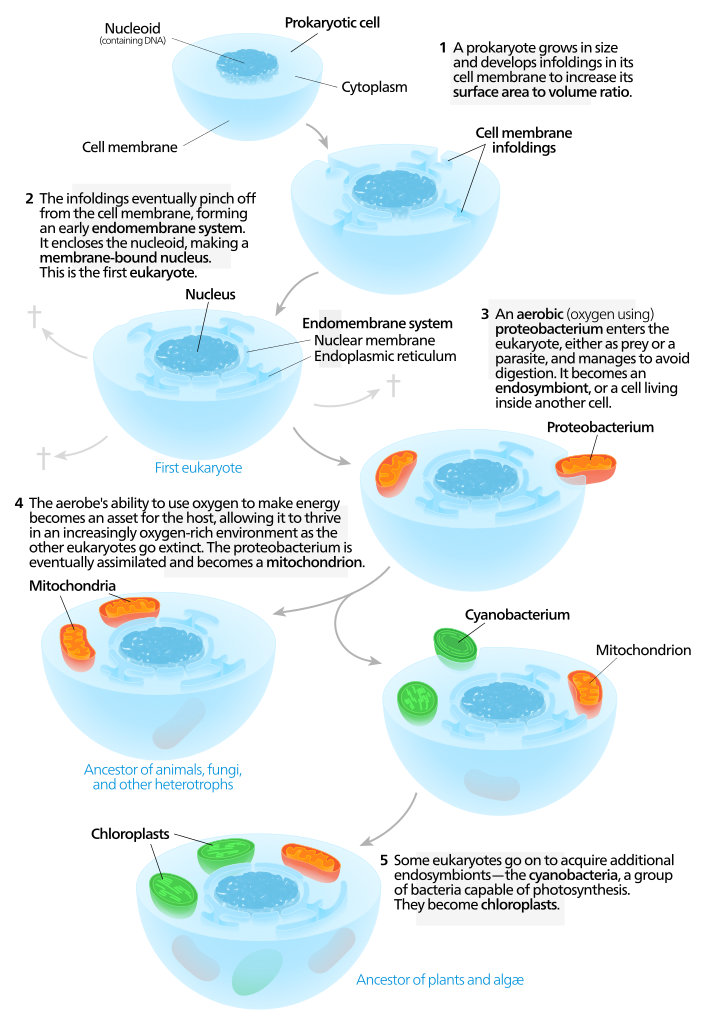
Attribution: Kelvinsong, CC BY-SA 3.0 , via Wikimedia Commons
Evidence That Chloroplasts Were Originally Cyanobacteria
Fig. 4 shows the similarities between a modern chloroplast and cyanobacterium, which have in common:
- DNA
- a double membrane,
- ribosomes (where mRNA is translated into amino acid sequences),
- thylakoids (where chlorophyll is found and the light-dependent reactions of photosynthesis occur)
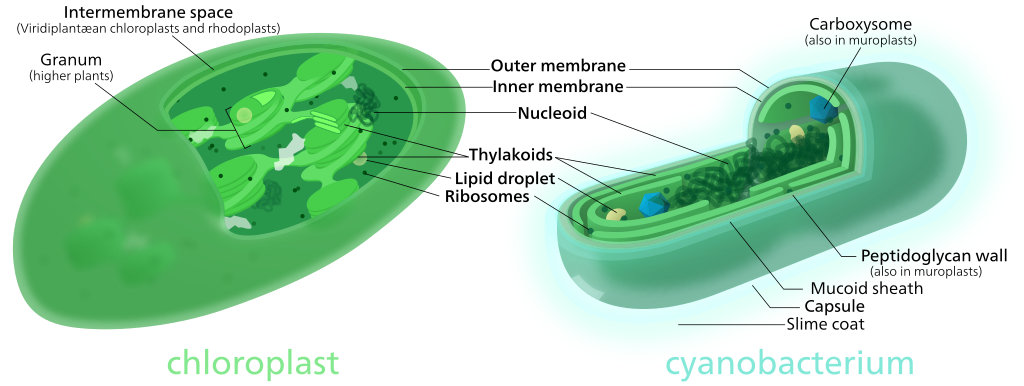
Attribution: Kelvinsong, CC BY-SA 3.0 , via Wikimedia Commons
Going further, cyanobacteria are the only photosynthesising prokaryotes with thylakoids, and by association, the only prokaryotes with chlorophyll. Similarly, chloroplasts are the only organelles with thylakoids and chlorophyll.
There is further evidence that chloroplasts evolved from a cyanobacterial ancestor, and much of this evidence also supports mitochondria evolving from an aerobic prokaryotic ancestor.
Chloroplasts (and mitochondria) are about the size of bacteria: 1 – 10 μm.
Not only do chloroplasts (and mitochondria) have their own DNA molecules, but these molecules are circular as is found in prokaryotes and not eukaryotes. (Chloroplast DNA is written ctDNA and mitochondrial DNA is written mtDNA.)
Another major piece of evidence is that chloroplasts (and mitochondria) reproduce themselves, and by binary fission, or cell division, just as prokaryotes do. If chloroplasts (and mitochondria) are removed from a cell, that cell cannot generate new chloroplasts (or mitochondria).
There is a close relationship between the chloroplast and cyanobacterial genomes. (Similarly, there is a close relationship between mitochondria and the bacterial genus Rikettsia.)
Many bacterial genes are missing in chloroplasts (and mitochondria). Some of these genes may have been lost over millenia, but the remainder are found in the nuclear chromosomes of their host eukaryote. This is indicative of a gene transfer having taken place.
Chloroplast (and mitochondrial) ribosomes are more like the ribosomes of bacteria than the ribosomes of eukaryotes.
Chloroplasts (and mitochondria) additionally have porins, proteins which aid in the transport of molecules across a cell membrane. Porins are only found elsewhere in some bacterial groups, including the cyanobacteria.
When Did the Chloroplast First Appear?
Cyanobacteria are believed to have first appeared around 2 – 2.4 billion years ago, when oxygen levels began to rise in the atmosphere from their photosynthetic activity.
The fossil record shows that eukaryotes had appeared by at least 1.5 billion years ago, and the symbiosis of a cyanobacterium with a eukaryote is believed to have occurred between 1 – 2 billion years ago.
The first photosynthesising eukaryotes were the red and brown algae, which first appeared 1.2 billion years ago. This suggests that the endosymbiont cyanobacterium had evolved into a chloroplast by this time.
The Significance of Endosymbiosis
Energy Source
All life needs an energy source. Those organisms which obtain theirs from the photons in sunlight are phototrophs. Those which obtain theirs by breaking chemical bonds are chemotrophs. Some, like plants, can do both!
This energy is converted into potential energy which is stored in a molecule called adenosine triphosphate (ATP). All life, without exception, no matter its evolutionary age or size, stores potential energy as ATP.
Electron Source
All life additionally needs an electron source (also called an electron donor source). Some, the lithotrophs, obtain theirs from inorganic materials such as hydrogen sulfide or ammonia. The organotrophs obtain theirs from organic materials such as carbohydrates and fats. Some, like plants again, can do both.
Those electrons move from the electron donor (ammonia, carbohydrate) to an electron acceptor, and this initiates chemical reactions to generate ATP.
Anaerobic Electron Acceptors
All life prior to the appearance of cyanobacteria was microbial and anaerobic (not requiring oxygen), as there was very little oxygen gas present in Earth’s atmosphere at the time. These are the anaerobes. Such organisms used (and still use) iron, nitrate, sulfate, sulfur, manganese, carbon dioxide and other substances as electron acceptors.
These elements and compounds, however, have low affinities for electrons compared to oxygen, and this limits the generation of ATP molecules. Anaerobic organisms can never generate enough potential energy to be anything other than single-celled organisms.
Cyanobacteria
The arrival of cyanobacteria changed the course of evolution drastically. These were the first phototrophs to use water as an electron source, which was split into hydrogen and oxygen ions. These oxygen ions readily formed oxygen gas. This production of oxygen as a by-product of cyanobacterial photosynthesis led to the eventual oxygenation of Earth, and cyanobacteria are the sole reason Earth’s atmosphere changed from less than 1% oxygen to 21% today.
This increase in oxygen led to the arrival of aerobes able to take advantage of it. Oxygen is a very strong electron acceptor and enables much larger quantities of ATP to be generated. The numbers differ depending on the specific metabolic pathway, but as an example, an anaerobe can only generate two molecules of ATP for every molecule of glucose, while an aerobe can generate 30 – 32 molecules. (The theoretical yield calculates as 38, but there are real-life losses to do with movement of intermediate molecules along the way.)
The Rise of Aerobes
Where it not for cyanobacteria, life may well have remained fully anaerobic — and microscopic — to this day. Instead, somewhere along the way, an aerobic prokaryote became engulfed by an early eukaryote, which itself was probably anaerobic. The union proved advantageous in that the prokaryote survived, and the eukaryote became better able to survive in an ever-increasingly oxygenated world. (Oxygen is toxic for many anaerobes.) The eukaryote became aerobic and the prokaryote became assimilated over time to become the first mitochondrion.
When a second prokaryote — a cyanobacterium — also became engulfed and assimilated to be the first chloroplast, the beginnings of macro life could begin in earnest, all fuelled by mitochondrial ATP and chloroplastic chlorophyll. And this is the world we are part of today, all because one cyanobacterium one day long ago was not digested!
About the Author

BSc(Hons), U.Syd. - double major in biochemistry and microbiology, with honours in microbiology
PhD, U.Syd - soil microbiology
Stumbled into IT and publishing of all things.
Discovered jujube trees and realised that perhaps I should have been an agronomist...
So I combined all the above passions and interests into this website and its blog and manuals, on which I write about botany, soil chemistry, soil microbiology and biochemistry - and yes, jujubes too!
Please help me buy a plant if you found this article interesting or useful!