From Soil to Fruit
- To Fruit
- Photosynthesis in Plants
- The Chloroplast
- Light-Dependent Reactions
- Electron Flow in Photosystem I (PSI)
Electron Flow in Photosystem I (PSI)
Electron Flow
All life is driven by electron flows through incredibly involved pathways called ‘electron transport chains’. (That it happens at all is just awe-inspiring!)
All such pathways begin with a primary electron donor and end with a terminal electron acceptor. It is the flow of electrons from the first donor to the last acceptor which drives all aspects of life.
Photosynthesis is just one example of an electron transport chain, of which there are different versions. The electron transport chain which is the oxygenic (oxygenic-producing) photosynthesis of plants, algae and cyanobacteria is very different to those of non-oxygenic photosynthesising bacteria.
Fig. 1 below represents the membrane of a thylakoid, found in all oxygenic photosynthesisers. In it are embedded four major protein complexes, and electrons must flow through the first three for photosynthesis to occur at all.
Electron flow — the electron transport chain — of oxygenic photosynthesis is represented by the cyan ‘e-’ circles:
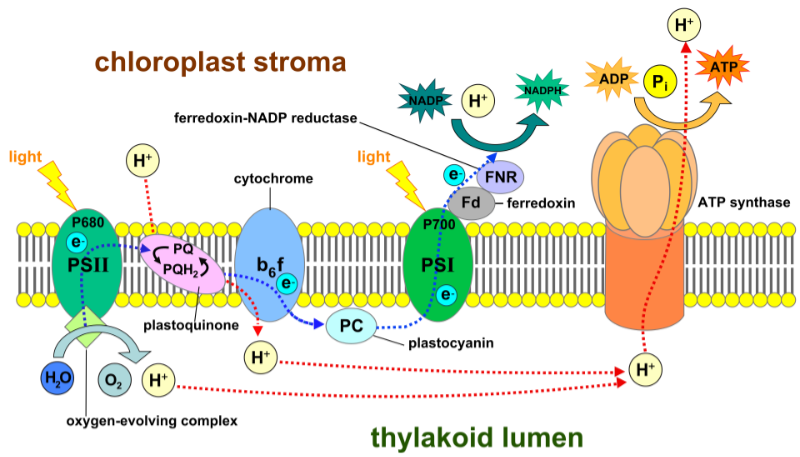
Attribution: By Somepics - Own work, CC BY-SA 4.0, https://commons.wikimedia.org/w/index.php?curid=38088695
Overview of Electron Flow From Photosystem II to Cytochrome b6f and Plastocyanin
Photosystem II (PSII)
Electron flow begins in the first major protein complex of photosynthesis: photosystem II (PSII). The high energy of light photons stimulates an electron in a molecule within PSII called P680. This electron reaches such a highly excited state that it is ejected — P680 is thus the primary electron donor.
This electron is replaced with one from the splitting of water elsewhere in PSII. Splitting water produces oxygen gas (O2, hence ‘oxygenic photosynthesis’).
A highly excited electron is a highly reactive one, and the ejected electron is readily taken up by a molecule called pheophytin. Pheophytin is the first electron acceptor.
Pheophytin, now highly reactive itself, passes that electron to another molecule, plastoquinone (PQ in Fig. 1 above). Pheophytin has become the second electron donor, and plastoquinone is now the second electron acceptor.
And so begins the electron flow from donor to acceptor, to donor, to acceptor, until the final acceptor is reached.
Between PSII and Cytochrome b6f
Plastoquinone, on acquiring a second electron from pheophytin, attracts two protons (hydrogen ions, H+) from outside the thylakoid, and becomes plastoquinol (PQH2 in Fig. 1 above). Plastoquinol leaves PSII, carrying two electrons and two protons, and enters the thylakoid membrane.
Plastoquinol is the means by which electrons move, via the thylakoid membrane, from the first protein complex (PSII) to the second protein complex (cytochrome b6f).
Cytochrome b6f
Cytochrome b6f is an enzyme. An enzyme is a protein which is a catalyst, or something which lowers the activation energy required for a reaction to proceed.
(Many, many biological reactions in all life would never happen at all where it not for catalysts, as more energy is needed than is available to the system. A catalyst lowers the energy required to make a reaction possible.)
Cytochrome b6f has two roles. One is to catalyse the transfer of electrons from plastoquinol to yet another molecule, plastocyanin (PC in Fig. 1 above). Transferring those two electrons releases the two protons acquired earlier by plastoquinol from outside the thylakoid, and cytochrome b6f’s second role is to pump these into the inside of the thylakoid. (These protons are now in place to be used by the fourth protein complex, ATP synthase, which we’ll cover in a later chapter.)
Between Cytochrome b6f and PSI
Plastocyanin’s role is to transfer the two electrons given it via cytochrome b6f to the third major protein complex in photosynthesis: photosystem I (PSI).
Electron Flow in Photosynthesis So Far
So far, we’ve covered the electron transport chain from PSII to just before photosystem I (PSI). The complex flow of electrons to this point has resulted in three very important events.
One is the splitting of water and production of oxygen gas, the sole means by which Earth’s atmosphere changed from 0% to 21% oxygen over two billion years or so, and why all macro-organisms including ourselves exist at all. (Only oxygen can provide the energy needed to sustain the electron transport chains of larger lifeforms.)
The second is the transfer of protons (hydrogen ions, H+) across the thylakoid membrane from the outside stroma to the inside lumen. This is a vital step in the production of energy called adenosine triphosphate (ATP), the universal energy needed by all life for metabolism, and we’ll cover this elsewhere.
While these two events are coupled to electron flow, the third involves those very electrons directly.
Those electrons, which were originally released from PSII by photon energy, were transported through the thylakoid membrane via several transport/carrier molecules, and now reside in plastocyanin, en route to PSI. They were carried all this way for one very important reason — to produce the electron-carrier molecule called nicotinamide adenine dinucleotide phosphate (NADPH). NADPH is the terminal electron acceptor in photosynthesis.
Electron Flow in Photosystem I (PSI)
Another name for photosystem I (PSI) is plastocyanin–ferredoxin oxidoreductase. Just as we broke down the alternative name for cytochrome b6f, plastoquinol-plastocyanin reductase, so too can we here, and know immediately how PSI functions!
The -ase ending signifies an enzyme, so immediately we know PSI is an enzyme. An oxidoreductase enzyme is one which transfers electrons from a donor (which is oxidised) to an acceptor (which is reduced). With this naming convention, the donor is listed first and the acceptor second. Thus PSI is an enzyme which facilitates the transfer of electrons from plastocyanin the donor, to ferredoxin the acceptor.
Ferredoxins are iron-sulfur proteins and amongst the most reducing of all biological electron carriers. To be reduced means to receive electrons, and it makes sense that such compounds are towards the end of a transport chain.
Let’s backtrack a bit and recall that PSII contains a chlorophyll-protein reaction centre called P680, and that PSI contains a reaction centre called P700. ‘P’ stands for ‘pigment, and the number refers to the wavelength of maximum absorption by that pigment.
P680 in PSII absorbs photon energy and kickstarts electron flow in photosynthesis by using that energy to donate an electron to pheophytin.
P700 in PSI also absorbs photon energy, but uses that energy to catalyse the transfer of electrons from plastocyanin to ferredoxin (Fd in Fig. 1 above). These electrons move across the thylakoid membrane, from the inside to the outside. Here, in the aqueous stroma of the chloroplast, those electrons are about to end their journey which began back in PSII.
Here in the stroma, ferredoxin, via the enzyme ferredoxin–NADP+ reductase (FNR in Fig. 1 above) donates those electrons to NADP+, which reduces to NADPH, the terminal electron acceptor in photosynthesis.
NADP+/NADPH
NADPH is the reduced form of NADP+, or nicotinamide adenine dinucleotide phosphate. The two work together to transfer electrons and protons (hydrogen ions, H+) to and from the enzymes needed to drive many anabolic biochemical reactions. This makes each a cofactor, or ‘helper molecule’.
(An anabolic reaction is one in which a larger molecule is synthesised from smaller ones — plants building carbohydrates from carbon dioxide is one such example where NADP+/NADPH are involved.)
NADP+/NADPH are universal electron carriers and found in all life.
NADP+ is an electron and proton acceptor (acquires electrons and H+) — accepting electrons and protons converts it into NADPH.
NADPH is an electron and proton donor (loses electrons and H+) — donating electrons and protons converts it into NADP+.
The End Result
Electron flow in photosynthesis (and all biological pathways for that matter) is incredibly involved, and reliant on complex molecules acting in turn to donate and accept electrons.
It is important to realise that this flow would not occur spontaneously in nature as the energy required is too great. Just as paper won’t spontaneously ignite, but needs help, electron flow in photosynthesis too requires help, and this is done in two ways.
One is to input energy into the system directly — just as inputting heat energy to paper causes ignition — and in photosynthesis this is done with two photon boosts, one in PSII and one in PSI.
The second form of help is to lower the energy required, and this is done via the enzyme activity within PSII, cytochrome b6f, and PSI.
Electron flow in oxygenic photosynthesis results in the splitting of water to produce oxygen gas as mentioned above. While this is a nice side-benefit for the rest of the planet, this gas production is actually a waste product which doesn’t affect the photosynthesiser directly.
The real purpose of electron flow in photosynthesis — from the photosynthesiser’s point of view — is to achieve two very important results.
One, as we’ve seen, is for electrons to end up in NADPH, the universal electron carrier and proton donor. NADPH, along with its NADP+ counterpart, goes on to participate in the Calvin Cycle, the means by which the carbon in carbon dioxide is ‘fixed’ into the carbon of glucose. (These compounds feature in other biochemical pathways as well.)
The second result of electron flow in photosynthesis is to pump protons (hydrogen ions, H+) across the thylakoid membrane, from the outside stroma to the inside lumen. Here they are available to participate in the second end result of photosynthesis, the generation of the energy molecule ATP. And it is this which we will cover in the next chapter.
About the Author

BSc(Hons), U.Syd. - double major in biochemistry and microbiology, with honours in microbiology
PhD, U.Syd - soil microbiology
Stumbled into IT and publishing of all things.
Discovered jujube trees and realised that perhaps I should have been an agronomist...
So I combined all the above passions and interests into this website and its blog and manuals, on which I write about botany, soil chemistry, soil microbiology and biochemistry - and yes, jujubes too!
Please help me buy a plant if you found this article interesting or useful!