What Makes Water So Important to Life?
Did you know there is only one substance that absolutely must be available to all living things on planet Earth?
It isn’t oxygen — while that’s essential for animals and for non-photosynthetic processes within a plant, it’s a poisonous gas to many microbes.
It isn’t a particular vitamin, like, say vitamin C is for us — many organisms can make their own and many other important compounds.
The one essential thing required by all life as we know it, to the extent that NASA in its search for extraterrestrial life skips over planets without it, is…water.
But what is it about water that makes it so crucial for life?
Its structure.
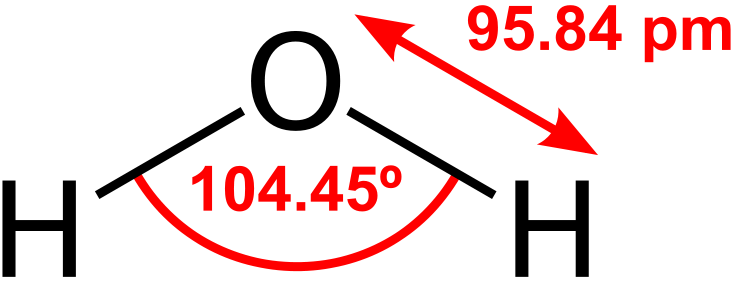
Attribution: Dan Craggs [Public domain]
(’pm’ stands for picometre, which is one-trillionth of a metre).
Most people would know that water is a molecule of one oxygen (O) atom and two hydrogen (H) atoms (hence the ‘H2O’ everyone is familiar with). But individual atoms, like O and H, have a neutral charge, and so to form reactions with each other to make molecules, they must first be in ionic form, with either a positive or negative charge overall. A positive charge results when there are more protons (+) than electrons (-), and a negative charge results when there are more electrons (-) than protons (+) in an ion.
A hydrogen ion has a charge of +1 (written as H+), which means it has one more proton than electron (in fact, a hydrogen ion is unique in that it has no electron). An oxygen ion has a charge of -2 (written as O2-), which means it has two less protons than electrons. Two hydrogen ions have a combined charge of +2, which is why a single oxygen ion attracts two hydrogen ions. Each of the oxygen ion’s ‘extra’ electrons bonds with one hydrogen ion, to make a neutrally-charged water molecule. We call the now molecular O and H here atoms again, and not ions, as once they are bonded they again have a neutral charge.
That explains the composition of a water molecule, but it doesn’t explain its structure. Why is the molecule bent, and not in a straight H-O-H arrangement?
While the oxygen atom in a water molecule has electrons bonded to hydrogen atoms, it also has two pairs of unbonded electrons opposite the two hydrogen atoms (to explain why this is so is quite involved and well beyond the scope of this post):
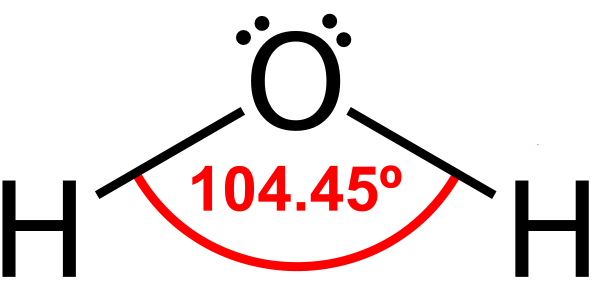
Modified image, based on previous image by Dan Craggs [Public domain]
If it weren’t for the two electron pairs, water would be a linear molecule, with a hydrogen-oxygen-hydrogen bond arrangement of 180°. Instead, the two electron pairs exert a force that pushes each oxygen-hydrogen bond away to cause the roughly 104° bend between them.
The diagram above is a simple two-dimensional representation of what is really a tetrahedral shape — as shown in the second image on this page. There is a slightly negative charge (written as δ-) near the two electron pairs, from the electrons themselves, and a slightly positive charge (written as δ+) near the hydrogen atoms, due to their single proton each. This uneven distribution of electron density (more electrons in the electron pairs than in the hydrogen atoms) not only bends the molecule, but makes it polar. That is, it has a negative pole at the oxygen end and a positive pole at the hydrogen end.
The bend is a consequence of water’s polarity, but it’s both the bend and the polarity that makes water essential to life. Let’s investigate further.
Water’s polarity makes hydrogen bonding possible. This is where the slightly positive hydrogen ends of water molecules are attracted to the slightly negative oxygen ends of other water molecules. This attraction is strong, and brings the molecules closer together than otherwise without this bonding. Crucially, the bend makes this attraction even stronger, by enabling the oxygen and hydrogen atoms of different molecules to be in closer proximity than if they were in a linear arrangement:
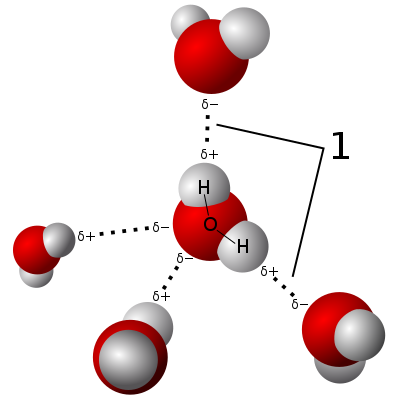
Attribution: User Qwerter at Czech wikipedia: Qwerter. Transferred from cs.wikipedia to Commons by sevela.p. Translated to English by Michal Maňas (User:snek01). Vectorized by Magasjukur2 [CC BY-SA 3.0 (https://creativecommons.org/licenses/by-sa/3.0)]
Most molecules with a similar mass to water (for example atmospheric nitrogen, N2) are gases at room temperature. The individual molecules of nitrogen gas, for example, are at too high a level of excitation to come together and form a liquid. It takes a temperature of at least -196 °C to slow them down enough that they do form liquid nitrogen!
Water molecules are not only prevented from bouncing around in a gaseous state because of hydrogen bonding, but the presence of two hydrogen atoms for every one oxygen atom means each water molecule can bond with two others, making for a stronger liquid state than if a one to one ratio.
The importance of hydrogen bonding holding water molecules together in a strong liquid formation cannot be overstated: it means water evaporates at a higher temperature than otherwise, it means water has surface tension and can cling to surfaces and move due to capillary action, and it means water is slow to heat up. It ultimately means that water is a liquid over a much wider range of temperatures than would be possible in the absence of hydrogen bonding. This in turn means that life can exist across the temperature extremes that exist naturally throughout days, seasons and across the greater Earth.
And water is not just any liquid, but a liquid so special that it is often referred to as the universal solvent. This means that it can dissolve nearly anything because of its polar nature. The negative oxygen ends of water molecules don’t just attract positive hydrogen ends, they can attract (hold in the water, dissolve) many positive ions you’d recognise as plant nutrients, such as sodium (Na+), potassium (K+), calcium (Ca+) and magnesium (Mg2+). Similarly, the positive hydrogen ends enable many negative ionic nutrients such as phosphates (PO43-, HPO42- and H2PO4-), sulfate (SO42-), and bicarbonate (HCO3-) to dissolve in water.
In short, water’s structure is crucial to life because it enables water to flow (being a fluid) and carry (being a solvent) over a wide range of temperatures. It can flow on and into land, and collect into rivers, lakes and oceans where it becomes available to organisms. Water in an organism moves in and out of cells, delivering nutrients essential in, and removing wastes from, biochemical reactions.
No other substance has all the properties of water that life as we know it depends on, which is why water has no substitute. Some other compound may be polar for example, but be a gas or a solid at the temperatures water is liquid at — no good if it solidifies overnight when temperatures drop, and has evaporated by mid-afternoon!
Let’s compare ethanol with water to make this point. Ethanol is polar, freezes at -114 °C, and boils (evaporates) at 78 °C. Plants wouldn’t need to enter dormancy during freezing winter temperatures if they used ethanol!
But this is where water’s other properties become so important. Ethanol’s lower boiling point of 78 °C would make life harder in warm climates and especially so in hot summers. Water, being harder to heat up and turn to gas, is an excellent coolant and makes life possible in such areas.
Hydrogen bonding in ethanol is also much weaker than in water, because of its size, its linear structure, and having only one hydrogen available to bond with any other oxygen.
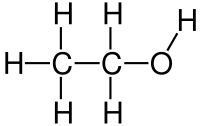
Attribution: Jü [Public domain]
This weaker hydrogen bonding significantly affects the dissolution of nutrients and lowers surface tension. Plants especially would struggle in these conditions. And while water pre-existed life on this planet, ethanol, as far as I can tell (and I’m happy to be corrected, and to update this post accordingly), is not made naturally via non-biochemical means. In other words, water jump-started life (notwithstanding the water paradox!), but life jump-started ethanol (via fermentation).
(Theorising alternatives to water for life, whether here or on other planets, is a fascinating topic that has preoccupied scientists and science-fiction writers for quite a while. Please look up ‘hypothetical types of biochemistry’ elsewhere should you wish to explore this further.)
Water not only ticks all the necessary boxes for life as we know it, it also enables life to extend into just about any extreme on Earth imaginable. Boiling hot thermal springs? No problem!
It is quite remarkable that such a simple little molecule has the indispensable properties it has — as hubby said, you couldn’t design a setup like that if you tried!
About the Author

BSc(Hons), U.Syd. - double major in biochemistry and microbiology, with honours in microbiology
PhD, U.Syd - soil microbiology
Stumbled into IT and publishing of all things.
Discovered jujube trees and realised that perhaps I should have been an agronomist...
So I combined all the above passions and interests into this website and its blog and manuals, on which I write about botany, soil chemistry, soil microbiology and biochemistry - and yes, jujubes too!
Please help me buy a plant if you found this article interesting or useful!