ATP (Adenosine Triphosphate) Synthase
A Summary of Oxygenic Photosynthesis to Date
Oxygenic photosynthesis produces oxygen gas (O2), and is performed by plants, algae and cyanobacteria. (Many other bacteria can photosynthesise, but these are anoxygenic photosynthesisers, typically producing pure sulfur as a product.)
Plants, algae and cyanobacteria are the only organisms with structures known as thylakoids, and it is in the thylakoid membrane that oxygenic photosynthesis occurs.
Fig. 1 below represents the thylakoid membrane, and the four major protein complexes within it that are involved in oxygenic photosynthesis:
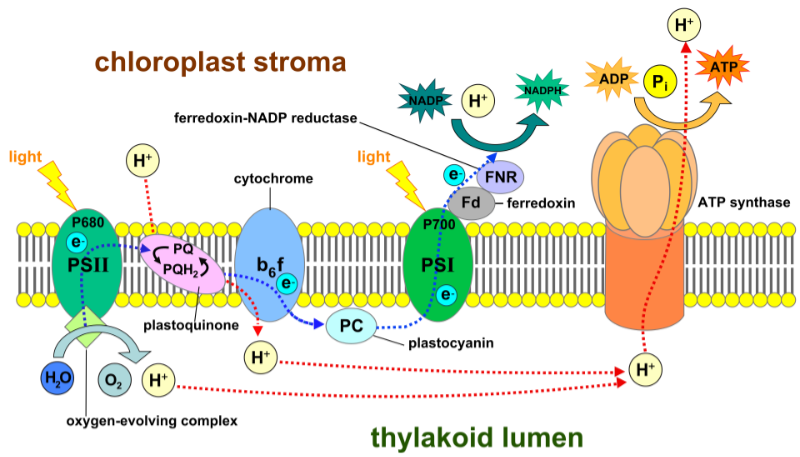
Attribution: By Somepics - Own work, CC BY-SA 4.0, https://commons.wikimedia.org/w/index.php?curid=38088695
So far, we’ve covered photosystem II (PSII), cytochrome b6f, photosystem I (PSI), and the electron flows through each of PSII and PSI.
This chapter discusses the fourth and final protein complex: ATP synthase.
ATP Synthase
Let’s break down the meaning of ‘ATP synthase’. ATP stands for adenosine triphosphate. The -ase ending of ’synthase’ tells us that synthase is an enzyme, or protein catalyst. ‘Syn’ implies ’synthesis’, thus ATP synthase is a protein catalyst which synthesises ATP. ATP is the universal molecule of energy storage, as it is found in, and needed by, absolutely every living cell on the planet from the smallest bacterium to the largest trees and animals. Thus ATP synthases (structures differ from group to group) are also found in absolutely every living cell on the planet.
Location in All Life
Where ATP synthase is located in all organisms reveals some interesting support for the endosymbiotic theory of prokaryotic (bacterial and archaeal) cells forming a union with eukaryotic cells (everything else), which paved the way for higher, multicellular life to evolve.
Prokaryotes
All prokaryotes — the bacteria and archaea — are single-celled organisms. These single cells are bound by a cell membrane, in which their versions of ATP synthase are embedded.
Cyanobacteria
Cyanobacteria, being bacteria, also have ATP synthase in their cell membranes. They are also oxygenic photosynthesisers, which means they further have internal structures called thylakoids. Thylakoids too are membrane-bound, and as can be seen in Fig. 1 above, ATP synthase resides in those membranes too.
Eukaryotes
All eukaryotes, whether single-celled like yeasts or multicellular like plants and animals, contain mitochondria which have inner and outer membranes. The only place ATP synthase is found in non-photosynthesising eukaryotes is in the inner membranes of their mitochondria, which adds support to the theory that mitochondria were once prokaryotes which formed a symbiosis with eukaryotes. Eukaryotic cells cannot make mitochondria — mitochondria have their own DNA and are self-replicating — and cannot survive without mitochondria synthesising their ATP needs.
Algae and Plants
Algae and plants are the only photosynthesising eukaryotes, and the only eukaryotes to contain chloroplasts. Chloroplasts contain thylakoids, and just as with cyanobacteria, those thylakoid membranes are also the location of ATP synthase. And just as cyanobacteria have ATP synthase in both their cell membrane and thylakoid membranes, so too do plants and algae have ATP synthase in both their mitochondrial and their thylakoid membranes. Plants and algae cannot photosynthesise without chloroplasts, and cannot make their own chloroplasts just as they cannot make their own mitochondria. This ATP synthase connection adds to the evidence that chloroplasts were once cyanobacteria that formed an additional union with (some) eukaryotes that evolved into algae and plants.
ATP Production
ATP (adenosine triphosphate) forms when a phosphate molecule attaches to a molecule called ADP (adenosine diphosphate). This reaction does not occur spontaneously owing to the energy required, and in fact it is the reverse reaction which does occur naturally, in which ATP readily breaks down to ADP and a phosphate group. It is this reverse reaction, of ATP breaking down into ADP, that releases the energy for biochemical pathways to proceed.
Thus all living cells require a constant supply of ATP that breaks down into ADP to release energy, else they die. But as ATP does not form spontaneously, cells need help in overcoming the energy input barrier for it to form at all. They need a catalyst, or a substance which drives a chemical reaction by lowering the energy required. This catalyst is ATP synthase.
The Mechanism of ATP Synthase
As a catalyst, ATP synthase works by lowering the energy required for phosphate to join with ADP to form ATP. It does this by making use of a proton gradient across the membrane it is embedded in. This is the case for all ATP synthases, whether they are in bacterial cell membranes, mitochondrial inner membranes, or the thylakoid membranes of cyanobacteria and chloroplasts.
The protons, also called hydrogen ions (H+), accumulate in the inner side of a membrane via an electron transport chain. This gradient from a high concentration inside the membrane to a lower concentration outside the membrane creates electrical potential, and it is this energy difference causing protons to move through the membrane which powers ATP synthase and provides the energy needed for ATP to form.
ATP Synthase in Oxygenic Photosynthesis
The conversion of ADP to ATP is known as phosphorylation — whereby a phosphoryl group (an ion containing phosphate and oxygen) becomes attached to another molecule.
Photophosphorylation is the phosphorylation of ADP to ATP using the energy of photons from visible sunlight.
Those photons’ role was to initiate photosynthesis by starting an electron flow from photosystem II (PSII), through to cytochrome b6f, and on to photosystem I (PSI), where electron flow ends with the formation of NADPH (nicotinamide adenine dinucleotide phosphate).
Along the way, protons entered the inner side of the thylakoid membrane (called the lumen) — some from the splitting of water in PSII, and some via the actions of cytochrome b6f.
This creates a proton gradient, with a higher concentration in the lumen and a lower concentration on the other side of the thylakoid membrane, in the stroma. Protons are pumped from the lumen to the stroma, and the energy of this electrical potential powers the ATP synthase molecule which spans the membrane.
Where to After ATP Synthase
Up Until Now: Light-Dependent Reactions of Photosynthesis
All chapters on photosynthesis so far have covered the four major protein complexes embedded in the thylakoid membrane: photosystem II (PSII), cytochrome b6f, photosystem I (PSI), the electron flows through each of PSII and PSI, and finally this one on ATP synthase.
All the reactions in all these complexes resulted in the formation of two molecules: NADPH (the universal electron and proton transporter), and ATP (the universal energy storage molecule). All these reactions are collectively called the light-dependent reactions, so-called as they rely on photon input to occur.
These light-dependent reactions of photosynthesis end with electrons in NADPH via PSI, and with the photophosphorylation of ADP to ATP via ATP synthase.
Everything covered so far can be summarised with the following reaction:
2H2O + 2NADP+ + 3ADP + 3Pi → O2 + 2H+ + 2NADPH + 3ATP
where Pi signifies inorganic phosphate.
But photosynthesis is supposedly the production of food via sunlight, and where is that in the above equation?
Enter the light-independent reactions of photosynthesis!
After ATP Synthase: Light-Independent Reactions of Photosynthesis
The light-independent reactions of photosynthesis do not use a photon input, hence the name. Light-dependent reactions occur in the thylakoid membrane, but the light-independent ones occur outside the membrane, in the stroma.
These reactions use the NADPH and ATP made in the stroma via the light-dependent reactions to produce a carbohydrate called glyceraldehyde-3-phosphate (G3P) from carbon dioxide — this is the production of food via sunlight part, and is called the Calvin cycle.
The Calvin cycle will be covered over several more chapters, but for now can be summarised with this equation:
3CO2 + 6NADPH + 6H+ + 9ATP → glyceraldehyde-3-phosphate (G3P) + 6NADP+ + 9ADP + 3H2O + 8Pi
where Pi signifies inorganic phosphate and G3P enters other reactions to be converted into glucose.
About the Author

BSc(Hons), U.Syd. - double major in biochemistry and microbiology, with honours in microbiology
PhD, U.Syd - soil microbiology
Stumbled into IT and publishing of all things.
Discovered jujube trees and realised that perhaps I should have been an agronomist...
So I combined all the above passions and interests into this website and its blog and manuals, on which I write about botany, soil chemistry, soil microbiology and biochemistry - and yes, jujubes too!
Please help me buy a plant if you found this article interesting or useful!